What are the Differences Between Mainstream Supercapacitor Structure Models?
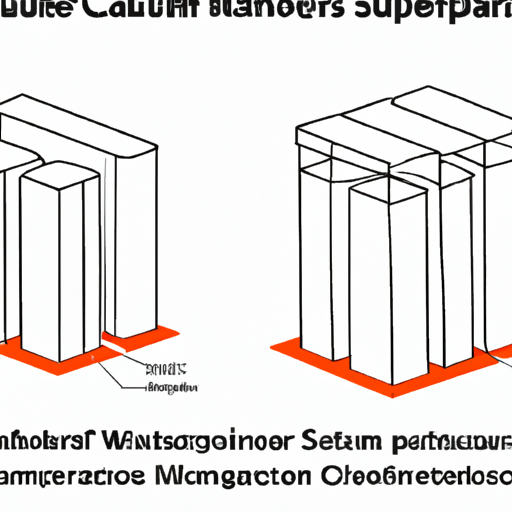
I. Introduction
Supercapacitors, also known as ultracapacitors or electrochemical capacitors, are energy storage devices that bridge the gap between traditional capacitors and batteries. They are characterized by their ability to store and release energy rapidly, making them essential in various applications, from consumer electronics to renewable energy systems. As the demand for efficient energy storage solutions continues to grow, understanding the different supercapacitor structure models becomes crucial for selecting the right technology for specific applications. This article aims to explore the differences between mainstream supercapacitor structure models, including Electric Double-Layer Capacitors (EDLCs), pseudocapacitors, and hybrid supercapacitors.
II. Basics of Supercapacitor Technology
A. Working Principle of Supercapacitors
Supercapacitors operate on two primary principles: electrostatic charge storage and electrochemical processes.
1. **Electrostatic Charge Storage**: Supercapacitors store energy through the separation of charges at the interface between the electrode and the electrolyte. This process occurs in the electric double layer formed at the surface of the electrodes.
2. **Electrochemical Double-Layer Capacitance (EDLC)**: In EDLCs, energy is stored in the electric field created by the separation of charges. The capacitance is determined by the surface area of the electrodes and the distance between the charges.
3. **Pseudocapacitance**: This phenomenon occurs in materials that undergo fast redox reactions, allowing for additional charge storage beyond the electric double layer. Pseudocapacitance can significantly enhance the energy density of supercapacitors.
B. Key Components of Supercapacitors
Supercapacitors consist of three main components:
1. **Electrodes**: Typically made from high-surface-area materials like activated carbon, graphene, or metal oxides, electrodes play a crucial role in determining the performance of supercapacitors.
2. **Electrolytes**: The electrolyte can be aqueous or organic, influencing the operating voltage and overall energy density of the supercapacitor.
3. **Separator**: This component prevents short circuits between the electrodes while allowing ionic transport, ensuring efficient charge storage and release.
III. Mainstream Supercapacitor Structure Models
A. Electric Double-Layer Capacitor (EDLC)
1. **Structure and Materials**: EDLCs utilize porous carbon-based materials for electrodes, which provide a large surface area for charge storage. The electrolyte can be either aqueous or organic, depending on the desired voltage range.
2. **Advantages and Limitations**: EDLCs are known for their high power density, long cycle life, and rapid charge/discharge capabilities. However, they typically have lower energy density compared to other types of supercapacitors, limiting their use in applications requiring high energy storage.
3. **Applications**: Common applications for EDLCs include regenerative braking systems in electric vehicles, backup power supplies, and energy storage in renewable energy systems.
B. Pseudocapacitor
1. **Structure and Materials**: Pseudocapacitors utilize materials that can undergo fast redox reactions, such as transition metal oxides or conducting polymers. This allows for additional charge storage mechanisms beyond the electric double layer.
2. **Advantages and Limitations**: Pseudocapacitors offer higher energy density compared to EDLCs, making them suitable for applications requiring more energy storage. However, they may have shorter cycle life and slower charge/discharge rates due to the electrochemical processes involved.
3. **Applications**: Pseudocapacitors are often used in applications like portable electronics, electric vehicles, and hybrid energy storage systems where higher energy density is essential.
C. Hybrid Supercapacitor
1. **Structure and Materials**: Hybrid supercapacitors combine the features of EDLCs and pseudocapacitors, typically using a combination of carbon-based materials and pseudocapacitive materials for the electrodes. This design aims to optimize both energy and power density.
2. **Advantages and Limitations**: Hybrid supercapacitors can achieve a balance between high energy density and power density, making them versatile for various applications. However, their complexity in design and manufacturing can lead to higher costs.
3. **Applications**: These supercapacitors are suitable for applications such as electric vehicles, grid energy storage, and renewable energy systems, where both energy and power density are critical.
IV. Comparative Analysis of Supercapacitor Models
A. Energy Density vs. Power Density
One of the primary differences between supercapacitor models lies in their energy and power density. EDLCs excel in power density, allowing for rapid charge and discharge cycles, while pseudocapacitors offer higher energy density, making them suitable for applications requiring sustained energy output. Hybrid supercapacitors aim to combine the best of both worlds, providing a balanced performance.
B. Charge/Discharge Cycles and Lifespan
EDLCs typically have a longer lifespan, with the ability to endure millions of charge/discharge cycles without significant degradation. Pseudocapacitors, while offering higher energy density, may experience a shorter lifespan due to the electrochemical reactions involved. Hybrid supercapacitors fall somewhere in between, with a lifespan that can vary based on the materials used.
C. Cost and Scalability
Cost is a significant factor in the adoption of supercapacitor technologies. EDLCs are generally more cost-effective due to their simpler manufacturing processes. Pseudocapacitors and hybrid supercapacitors, while offering enhanced performance, can be more expensive to produce. Scalability also varies, with EDLCs being easier to scale for large applications compared to the more complex designs of pseudocapacitors and hybrids.
D. Environmental Impact and Sustainability
The environmental impact of supercapacitors is an essential consideration. EDLCs, often made from activated carbon, can be produced sustainably, while pseudocapacitors may involve materials that are less environmentally friendly. Hybrid supercapacitors' sustainability depends on the materials used, making it crucial to consider the entire lifecycle of the device.
V. Emerging Trends and Innovations
A. Nanostructured Materials
Recent advancements in nanotechnology have led to the development of nanostructured materials that enhance the performance of supercapacitors. These materials can increase surface area, improve conductivity, and enhance charge storage capabilities.
B. Flexible and Wearable Supercapacitors
The demand for flexible and wearable electronics has spurred innovation in supercapacitor design. Researchers are developing lightweight, flexible supercapacitors that can be integrated into clothing and other wearable devices, providing convenient energy storage solutions.
C. Integration with Renewable Energy Sources
As the world shifts towards renewable energy, supercapacitors are being integrated with solar and wind energy systems to provide efficient energy storage and management solutions. This integration can help stabilize energy supply and improve the overall efficiency of renewable energy systems.
D. Future Directions in Supercapacitor Research
Future research in supercapacitor technology is likely to focus on improving energy density, reducing costs, and enhancing sustainability. Innovations in materials science, nanotechnology, and manufacturing processes will play a crucial role in advancing supercapacitor technology.
VI. Conclusion
In summary, the differences between mainstream supercapacitor structure models—EDLCs, pseudocapacitors, and hybrid supercapacitors—are significant and influence their performance, applications, and suitability for various energy storage needs. Understanding these differences is essential for selecting the right model for specific applications, whether it be for high power density, energy density, or a balance of both. As the demand for efficient energy storage solutions continues to grow, the future outlook for supercapacitor technology remains promising, with ongoing innovations and research paving the way for more advanced and sustainable energy storage solutions.
VII. References
A comprehensive list of academic papers, industry reports, and relevant books and resources would be included here to support the information presented in the article.
What are the Differences Between Mainstream Supercapacitor Structure Models?
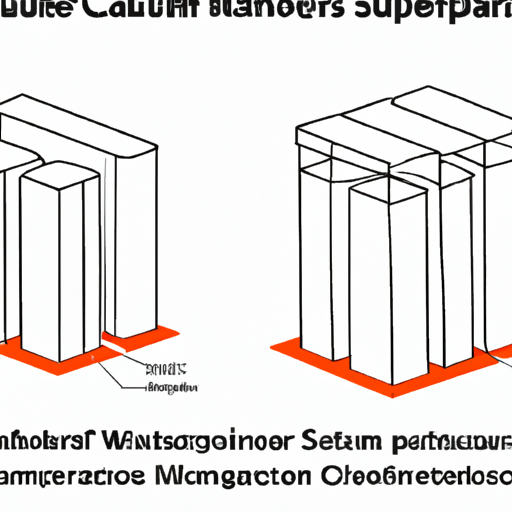
I. Introduction
Supercapacitors, also known as ultracapacitors or electrochemical capacitors, are energy storage devices that bridge the gap between traditional capacitors and batteries. They are characterized by their ability to store and release energy rapidly, making them essential in various applications, from consumer electronics to renewable energy systems. As the demand for efficient energy storage solutions continues to grow, understanding the different supercapacitor structure models becomes crucial for selecting the right technology for specific applications. This article aims to explore the differences between mainstream supercapacitor structure models, including Electric Double-Layer Capacitors (EDLCs), pseudocapacitors, and hybrid supercapacitors.
II. Basics of Supercapacitor Technology
A. Working Principle of Supercapacitors
Supercapacitors operate on two primary principles: electrostatic charge storage and electrochemical processes.
1. **Electrostatic Charge Storage**: Supercapacitors store energy through the separation of charges at the interface between the electrode and the electrolyte. This process occurs in the electric double layer formed at the surface of the electrodes.
2. **Electrochemical Double-Layer Capacitance (EDLC)**: In EDLCs, energy is stored in the electric field created by the separation of charges. The capacitance is determined by the surface area of the electrodes and the distance between the charges.
3. **Pseudocapacitance**: This phenomenon occurs in materials that undergo fast redox reactions, allowing for additional charge storage beyond the electric double layer. Pseudocapacitance can significantly enhance the energy density of supercapacitors.
B. Key Components of Supercapacitors
Supercapacitors consist of three main components:
1. **Electrodes**: Typically made from high-surface-area materials like activated carbon, graphene, or metal oxides, electrodes play a crucial role in determining the performance of supercapacitors.
2. **Electrolytes**: The electrolyte can be aqueous or organic, influencing the operating voltage and overall energy density of the supercapacitor.
3. **Separator**: This component prevents short circuits between the electrodes while allowing ionic transport, ensuring efficient charge storage and release.
III. Mainstream Supercapacitor Structure Models
A. Electric Double-Layer Capacitor (EDLC)
1. **Structure and Materials**: EDLCs utilize porous carbon-based materials for electrodes, which provide a large surface area for charge storage. The electrolyte can be either aqueous or organic, depending on the desired voltage range.
2. **Advantages and Limitations**: EDLCs are known for their high power density, long cycle life, and rapid charge/discharge capabilities. However, they typically have lower energy density compared to other types of supercapacitors, limiting their use in applications requiring high energy storage.
3. **Applications**: Common applications for EDLCs include regenerative braking systems in electric vehicles, backup power supplies, and energy storage in renewable energy systems.
B. Pseudocapacitor
1. **Structure and Materials**: Pseudocapacitors utilize materials that can undergo fast redox reactions, such as transition metal oxides or conducting polymers. This allows for additional charge storage mechanisms beyond the electric double layer.
2. **Advantages and Limitations**: Pseudocapacitors offer higher energy density compared to EDLCs, making them suitable for applications requiring more energy storage. However, they may have shorter cycle life and slower charge/discharge rates due to the electrochemical processes involved.
3. **Applications**: Pseudocapacitors are often used in applications like portable electronics, electric vehicles, and hybrid energy storage systems where higher energy density is essential.
C. Hybrid Supercapacitor
1. **Structure and Materials**: Hybrid supercapacitors combine the features of EDLCs and pseudocapacitors, typically using a combination of carbon-based materials and pseudocapacitive materials for the electrodes. This design aims to optimize both energy and power density.
2. **Advantages and Limitations**: Hybrid supercapacitors can achieve a balance between high energy density and power density, making them versatile for various applications. However, their complexity in design and manufacturing can lead to higher costs.
3. **Applications**: These supercapacitors are suitable for applications such as electric vehicles, grid energy storage, and renewable energy systems, where both energy and power density are critical.
IV. Comparative Analysis of Supercapacitor Models
A. Energy Density vs. Power Density
One of the primary differences between supercapacitor models lies in their energy and power density. EDLCs excel in power density, allowing for rapid charge and discharge cycles, while pseudocapacitors offer higher energy density, making them suitable for applications requiring sustained energy output. Hybrid supercapacitors aim to combine the best of both worlds, providing a balanced performance.
B. Charge/Discharge Cycles and Lifespan
EDLCs typically have a longer lifespan, with the ability to endure millions of charge/discharge cycles without significant degradation. Pseudocapacitors, while offering higher energy density, may experience a shorter lifespan due to the electrochemical reactions involved. Hybrid supercapacitors fall somewhere in between, with a lifespan that can vary based on the materials used.
C. Cost and Scalability
Cost is a significant factor in the adoption of supercapacitor technologies. EDLCs are generally more cost-effective due to their simpler manufacturing processes. Pseudocapacitors and hybrid supercapacitors, while offering enhanced performance, can be more expensive to produce. Scalability also varies, with EDLCs being easier to scale for large applications compared to the more complex designs of pseudocapacitors and hybrids.
D. Environmental Impact and Sustainability
The environmental impact of supercapacitors is an essential consideration. EDLCs, often made from activated carbon, can be produced sustainably, while pseudocapacitors may involve materials that are less environmentally friendly. Hybrid supercapacitors' sustainability depends on the materials used, making it crucial to consider the entire lifecycle of the device.
V. Emerging Trends and Innovations
A. Nanostructured Materials
Recent advancements in nanotechnology have led to the development of nanostructured materials that enhance the performance of supercapacitors. These materials can increase surface area, improve conductivity, and enhance charge storage capabilities.
B. Flexible and Wearable Supercapacitors
The demand for flexible and wearable electronics has spurred innovation in supercapacitor design. Researchers are developing lightweight, flexible supercapacitors that can be integrated into clothing and other wearable devices, providing convenient energy storage solutions.
C. Integration with Renewable Energy Sources
As the world shifts towards renewable energy, supercapacitors are being integrated with solar and wind energy systems to provide efficient energy storage and management solutions. This integration can help stabilize energy supply and improve the overall efficiency of renewable energy systems.
D. Future Directions in Supercapacitor Research
Future research in supercapacitor technology is likely to focus on improving energy density, reducing costs, and enhancing sustainability. Innovations in materials science, nanotechnology, and manufacturing processes will play a crucial role in advancing supercapacitor technology.
VI. Conclusion
In summary, the differences between mainstream supercapacitor structure models—EDLCs, pseudocapacitors, and hybrid supercapacitors—are significant and influence their performance, applications, and suitability for various energy storage needs. Understanding these differences is essential for selecting the right model for specific applications, whether it be for high power density, energy density, or a balance of both. As the demand for efficient energy storage solutions continues to grow, the future outlook for supercapacitor technology remains promising, with ongoing innovations and research paving the way for more advanced and sustainable energy storage solutions.
VII. References
A comprehensive list of academic papers, industry reports, and relevant books and resources would be included here to support the information presented in the article.